The rest mass of ghost neutrinos is one of the most sought-after quantities in particle physics, and scientists are one step closer to an accurate determination thanks to a new experiment led by researchers from the Max Planck Institute for Nuclear Physics in Germany.
If the mass of the neutrino were known, it could open the door to physics beyond the Standard Model of particle physics, which describes all the known forces and particles in the universe.
To say that neutrinos are strange is an understatement. Once thought to have no mass at all, it is now clear that this barely existing particle is actually three types in one, with identities fluctuating in a strange quantum blur as they fly through space. This shimmering identity implies a mass that also has different shapes, smeared in the changing likeness of neutrinos.
Being so light and strange, neutrinos can play by different rules than other particles. Accurately piecing together strokes of its incredibly small masses may even help confirm or rule out new models in particle physics.
However, physicists cannot simply weigh clusters of stationary neutrinos like grapes on a scale. Instead, they can only confirm the existence of such subatomic particles by observing their interactions with other particles or measuring their decay products. A particle can only exist for the briefest of moments, but during that time it leaves a trace that physicists can use to determine its mass.
However, having no charge and practically no gravitational attraction, neutrinos exert only the weakest force on other particles. In fact, billions of neutrinos are passing through your body right now, most of them coming from the Sun, but they rarely interact with us.
However, just because neutrinos have so little effect on other subatomic particles does not mean that they are not a fundamental part of what matter is made of. These are the most common particles that have mass in the universe, and knowing what gives these neutrino variations such a tiny, non-zero rest mass could help physicists iron out or understand some of the contradictions in the Standard Model that neutrinos introduce into the way they oscillate.
Physicists are constantly improving their best estimates of upper bounds on individual and collective neutrino masses using various methods. The most accurate measurement of the "flavor" of the electron neutrino showed that its mass cannot be greater than 0,8 electron volts. Translating this to a relative mass of 1 kilogram (or 2,2 pounds), it is the same as the weight of four raisins relative to the Sun.
The latest estimate was made in February 2022 by the Karlsruhe Tritium Neutrino Experiment (KATRIN) in Germany, based on the sputtering of electrons and neutrinos released by the superheavy form of hydrogen decay.
Another way to determine the mass of a neutrino, albeit a small one, is to study what happens when the atomic nucleus of the artificial isotope holmium-163 absorbs an electron from its inner shell. As a result, a proton turns into a neutron, the element dysprosium-163 is formed and a neutrino is released.
Physicists can measure the total energy released in this decay, using a kind of calorimeter, and infer the mass of the "missing" neutrino that escaped into the ether, based on the total mass of the atom and Einstein's famous equation, E = mc2, where mass and energy are equivalent .
This is calculated as the so-called Q value: the difference in energy that can be translated into the mass "disappeared" from the total number of atomic particles after the decay reaction. This difference in mass is interpreted as a neutrino.
However, the gold atoms in which holmium-163 is embedded can influence this decay reaction, explains Christoph Schweiger, a physicist at the Max Planck Institute for Nuclear Physics and lead author of the new study.
"It is therefore important to measure the Q value as accurately as possible using an alternative method and compare it to the calorimetrically determined value to identify possible systematic sources of error," he says.
To do this, Schweiger and his colleagues set up an experiment that combined five so-called Penning traps, located one above the other inside a superconducting magnet placed in a vacuum and immersed in liquid helium at a temperature of about 4 degrees Kelvin (-269,1°C or - 452,5°F).
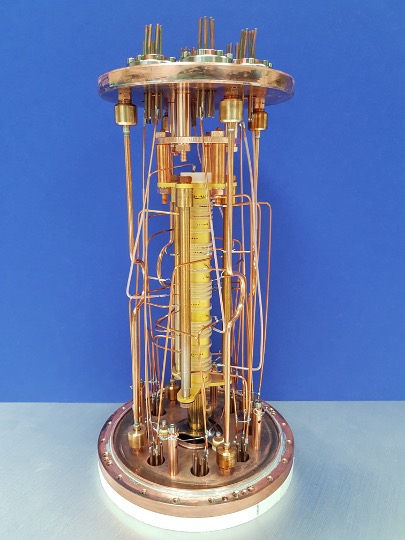
The PENTATRAP consists of five Penning traps stacked one above the other, as seen in the central yellow tower. (MPI in nuclear physics)
All these efforts help protect the equipment so that it is sensitive enough to capture particles in Penning traps and measure the subtle energy differences between the charged ions of holmium-163 and dysprosium-163.
"With the help of an Airbus A-380 with a maximum load, it would be possible to determine whether even a single drop of water fell on it," says Schweiger.
In fact, the researchers measured the incoming holmium-163 ions and the resulting dysprosium-163 ions to obtain a Q value of 2 ± 863,2 eV c-0,6 , which is 2 times more accurate than the previous study, which gave a value of 50 ± 2 eV c-833.
Using a more accurate and independently measured value of Q in conjunction with other experimental results "is vital to estimate the systematic uncertainties in neutrino mass determinations," Schweiger and his colleagues write in their published paper.
Although only one piece of the puzzle, improving the accuracy of measuring quantities like Q can be combined with a wide range of approaches to understanding why the strange, twinkling ghosts of the particle world behave the way they do.